Prof. Dr. Oliver Bruns
Department of Functional Imaging in Surgical Oncology, National Center for Tumor Diseases Dresden, Germany
Background
The lab of Dr. Oliver Bruns is dedicated to the development of imaging techniques and novel contrast agents in the short-wave infrared (SWIR) and near-infrared (NIR) range. This range uses wavelengths between 700 and 2000 nm (beyond the visible light range), in order to generate high-resolution in vivo images at depths not possible with conventional imaging.
Imaging at longer NIR and SWIR wavelengths can be very beneficial due to less scattering with thicker samples (such as tissue slices or whole animals), reduced autofluorescence, lower energies (less bleaching and photodamage allowing for longer experiments), and less absorption.
One issue with NIR imaging is a lack of usable probes or contrast agents at longer wavelengths, but the lab of Dr. Bruns continually develops new dyes with sensitivity in the NIR and SWIR, with these dyes and NIR and SWIR sensitive cameras, deep multicolor imaging can be performed in vivo on large samples.
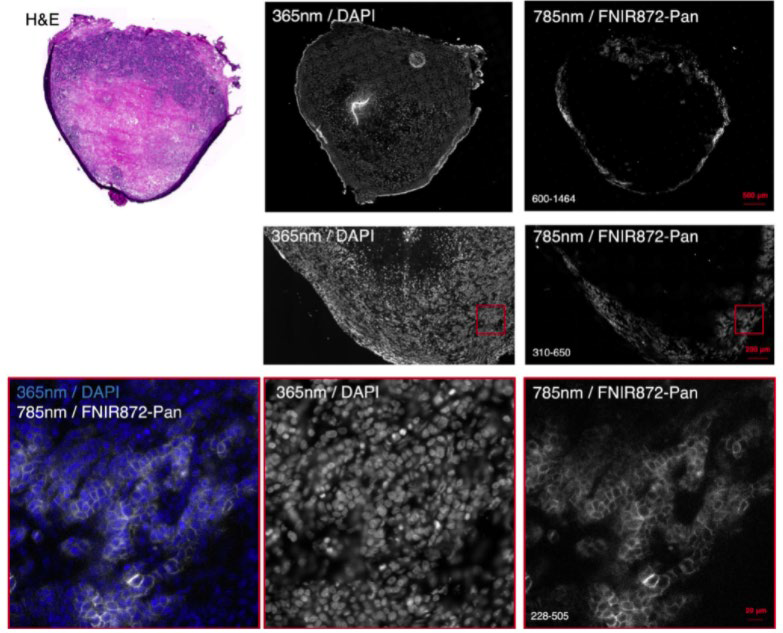
Challenge
Imaging at longer wavelengths can be challenging due to the fact that silicon is transparent at wavelengths longer than 1100 nm, with typical sCMOS cameras sharply dropping in sensitivity beyond 1000 nm. When using silicon-based sensors for NIR imaging, it is vital to have a broad quantum efficiency curve in order to retain sensitivity from 800 nm and above.
In addition, NIR imaging lends itself well to large, thick samples due to the penetrative ability to image at greater depth than visible imaging. This means that large samples are often used and a camera with a large field of view would be most suitable, in order to avoid excessive stitching and tiling to image in vivo samples.
Small pixel size is also desirable to allow for sub-cellular resolution across these larger images at optimized magnifications, allowing for high image quality at scale and fine details at smaller ROIs.
The [Prime BSI] has great sensitivity in the NIR around 900 nm, and performed well in our experiments.
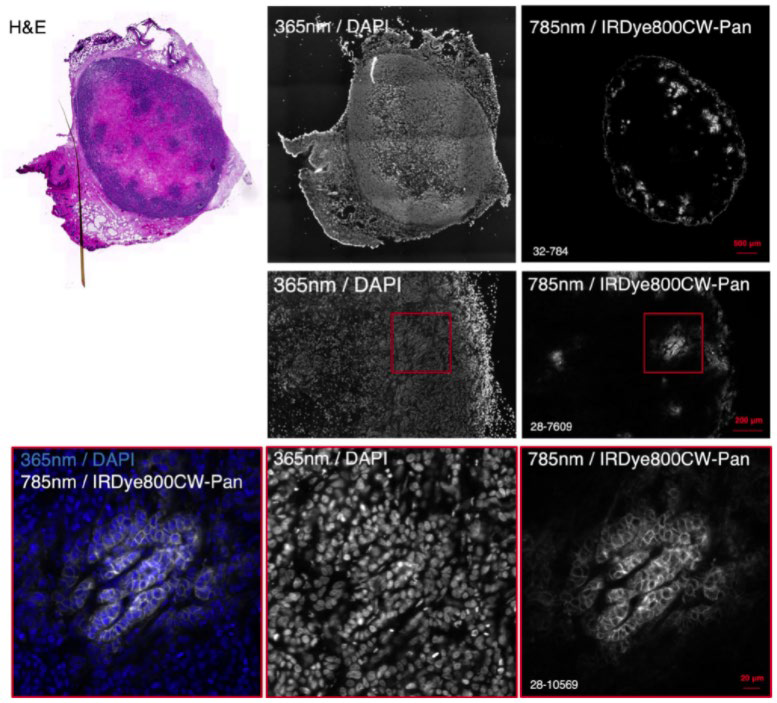
Solution
The Prime BSI features a broad quantum efficiency curve, with a peak of 95% and ranging from 200 nm to over 1000 nm, resulting in high sensitivity in the 700-900 nm NIR-I band. Combining this maximized signal collection with minimized noise thanks to the low-noise CMS mode of the Prime BSI results in a highly sensitive solution for NIR imaging.
The Prime BSI uses a small 6.5 μm pixel across a large 19 mm array, resulting in the combination of a large field of view while retaining high resolution at a range of magnifications.
Dr. Bruns recently used the Prime BSI for NIR imaging of murine tumor sections, using NIR dyes such as IRDye 800CW and FNIR-872 (emitting at around 900 nm) in combination with visible dye DAPI. These dyes were used for large format, high-resolution multicolor imaging across thick mouse organ sections in a recent Nature Methods publication.
Reference: Bandi, V. G., Luciano, M. P., Saccomano, M., Patel, N. L., Bischof, T. S., Lingg, J., Tsrunchev, P. T., Nix, M. N., Ruehle, B., Sanders, C., Riffle, L., Robinson, C. M., Difilippantonio, S., Kalen, J. D., Resch-Genger, U., Ivanic, J., Bruns, O. T., & Schnermann, M. J. (2022). Targeted multicolor in vivo imaging over 1,000 nm enabled by nonamethine cyanines. Nature methods, 10.1038/s41592-022-01394-6. Advance online publication. https://doi.org/10.1038/s41592-022-01394-6