Dr. Masayuki Mori
Kyoto University, Laboratory of Molecular Biology
Background
Calcium ion (Ca2+) is a second messenger involved in various physiological phenomena. In contrast to the high Ca2+ concentration (2 mM) in the extracellular fluid, cells maintain the cytosolic Ca2+at nM concentration. However, in the presence of external stimuli, the cytosolic Ca2+concentration increases approximately 100 times through the activation of calcium channels on the plasma membrane, and triggers a diverse array of physiological responses. In order to delineate the physiological significance of these calcium channels, the Laboratory of molecular Biology at Kyoto University studies voltage dependent calcium channels (VDCCs) and transient receptor potential (TRP) channels at various levels of an organism using molecular, cellular and physiological techniques.
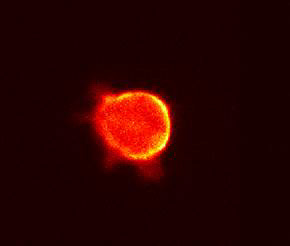

Challenge
The primary challenge in this research is the ability to capture simultaneous measurements of the ion channel activity by patch clamp and the FRET dynamics of lipid biosensor. A sophisticated imaging solution is essential.
The scientific imaging solutions from Teledyne Photometrics has made evolutional progress on our eletrophysiology and FRET research applications.
Solution
The Photometrics Evolve® 512 EMCCD camera (new series now available) and the DualView multichannel system were both demonstrated. After discussions with a knowledgeable imaging expert, the team selected these cameras as the imaging solutions that would support their research. Since implementing the cameras, they have found that their research has greatly improved with the ability to capture images with such high speed and low noise.
The team simultaneously and quantitatively measured the lipid dynamics by fluorescence changes and ionic flow from ion channels by combining with the patch-clamp. The fluorescence detection was completely dependent on the Evolve 512. “We have chosen this camera because of its high sensitivity, high temporal resolution, and low electrical noise,” states Dr. Mori. As the result, the membrane lipid component (PIP2) was clearly synchronized to the Ca2+ and Na+ flow to activate the cells. Dr. Mori concludes, “The scientific imaging solutions from Photometrics has made evolutional progress on our electrophysiology and FRET research applications.”

References
Recently published research by Dr. Mori and his team is accessible online at PubMed.gov: http://www.ncbi.nlm.nih.gov/pubmed/24470487