Professor Thorsten Hugel
PhD Candidate Leonie Vollmar
Institute of Physical Chemistry II, University of Freiburg, Germany
Background
The lab of Prof. Hugel is interested in obtaining real-time measurements of multi-protein complexes and their dynamic interaction. In living organisms, multi-protein complexes form intricate protein machines that regulate cellular processes. Of particular interest is the heat shock protein Hsp90 machinery, which consists of an Hsp90 dimer, co-chaperones, and client proteins. The complexes likely exist in multiple states that dynamically interchange and might be coordinated by utilizing the energy of ATP hydrolysis.
In the past, multicolor single-molecule FRET (Förster Resonance Energy Transfer) allowed Prof. Hugel and his team to study the Hsp90 machinery, determining the success of association and dissociation steps as well as large conformational changes of protein complexes simultaneously in real-time. Single-molecule FRET was also used to determine specific protein interfaces that interact as part of a complex. These studies impact the understanding of the Hsp90 machinery as well as general principles of multi-component protein systems, which is the basis for understanding cellular processes.
Challenge
Studying protein dynamics in real-time requires the ability to image on a wide range of timescales, meaning that any increase in imaging bandwidth would help to obtain a better understanding of protein interactions across all lengths of experiment.
Further challenges were outlined by Prof. Hugel: “For a proper dynamic analysis of several states of protein interaction many single-molecule FRET traces are needed, therefore an increased field of view (FOV) would help. As the analysis is carried out on a pixel-by-pixel basis a very high degree of stability, reliability and reproducibility are required, this can only be achieved by perfect noise characteristics of the imaging device”.
With a sensor diagonal of 18.6mm, the Prime 95B improved the FOV by a factor of >2.5x allowing for more data to be acquired at the same time
Solution
As Prof. Hugel states: “the Prime 95B seems to be a perfect answer to all questions asked, with a maximum speed of 82 fps it is significantly faster than standard EMCCD solutions, and without the need to crop the image.”
With a sensor diagonal of 18.6mm, the Prime 95B improved the FOV by a factor of >2.5x allowing for more data to be acquired at the same time. Prof Hugel mentioned that “the 11 x 11 μm pixel size enhances the resolution of the images compared to the previous EMCCD solution… this will accelerate the science by significantly strengthening the statistical validity.”
Prof. Hugel and his team are further optimizing the Prime 95B in their experiments, and have achieved a landmark experiment: “to our knowledge, this is the first time that dynamic single-molecule FRET traces of more than 10 seconds length were recorded on an sCMOS camera… there is a lot of potential, as a significantly higher time resolution is possible.”
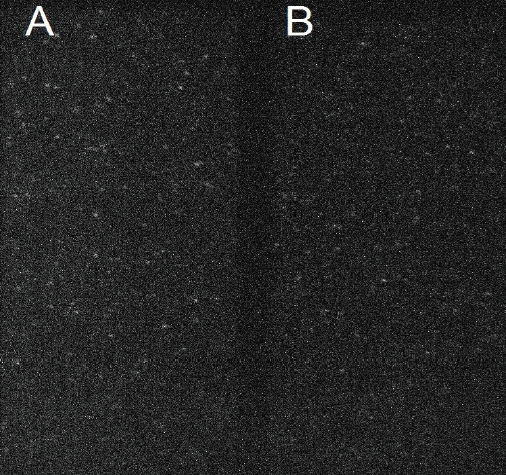

Holliday junctions recorded on the Prime 95B. Donor (green) and acceptor (red) fluorescence upon donor excitation in arbitrary digital units (ADU), uncorrected FRET efficiency (E, gray), Viterbi path by SMACKS1 (black). Exposure time 100 ms.
Additional Information
https://www.singlemolecule.uni-freiburg.de/
- S. Schmid, M. Götz, T. Hugel (2016) Single-molecule Analysis beyond Dwell Times: Demonstration and Assessment in and out of Equilibrium, Biophysical Journal 111, 1375-84