Professor Colin Brownlee, Marine Biological Association
Plymouth Director 2007-2017, Marine Biological Association Chair of Marine Biosciences, University of Southampton
Background
Phytoplankton play a critical role in Earth’s carbon and nutrient cycles, and in the regulation of our climate, yet are relatively poorly understood. The Brownlee Group at the Marine Biological Association study various processes that occur in living phytoplankton ranging from interactions between large populations of organisms to processes within single cells. Conventional microscopes and objectives are incapable of studying large enough populations of cells with sufficient resolution to discern sub-cellular details. The group therefore use the Mesolens system, a 4× objective with nearly 0.5NA, which can provide highly detailed images for a field of view 5mm across. The Mesolens, developed by Brad Amos and Gail McConnell at the University of Strathclyde, uses precision-made lenses to minimize spherical and chromatic aberration across the huge field of view.
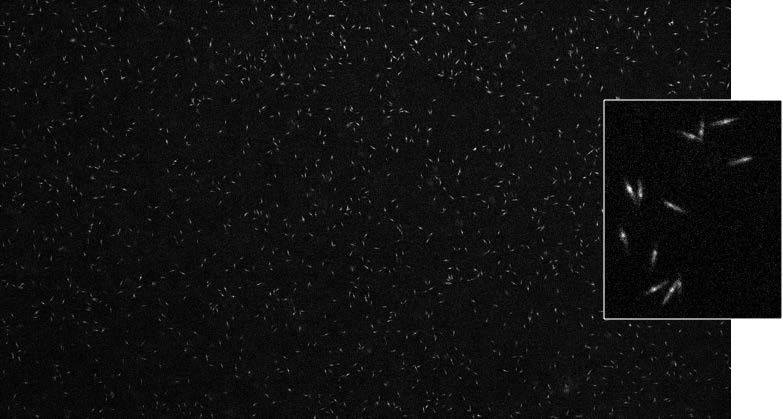
Challenge
The group are using calcium imaging to study dynamic behaviour in large populations of diatoms and coccolithophores expressing R-GECO, and need to preserve sub-cellular details and structures. A conventional CMOS camera wouldn’t have the field of view and resolution to take advantage of the Mesolens, so a moving-array CCD was previously used – however this camera was incapable of the sensitivity and speed required for calcium imaging.
The Iris 15 [Scientific CMOS camera] gives us the field of view, resolution and sensitivity to use calcium imaging to study interactions between large populations of cells with single-cell resolution on our Mesolens microscope.
Solution
The Photometrics Iris 15 Scientific CMOS (sCMOS) camera, with its 25mm field of view and 4.25µm pixels uniquely provides the field of view, resolution, sensitivity and speed for such detailed studies of large populations of cells. “One image shows us around a thousand cells,” says Professor Brownlee, Chair of Marine Biosciences, University of South Hampton. “We can capture a usable image with a 200ms exposure time on the Iris 15. The minimum exposure for a similar image from our previous camera was over a second – and then the camera would take two seconds to read the image out!”