Professor Andreas Mayer
Department of Biochemistry, Université de Lausanne, Switzerland
Background
Prof. Mayer and group work to study the cell membrane, especially the processes of membrane fusion and fission, exploring the molecular machinery that drives these reactions. Of particular interest are endosomes, lysosomes and other components of the endolysosomal system, which routinely fuse amongst each other to transfer cargo proteins or with the cell membrane to initiate repair and to release their contents out of the cell.
Most of this study is performed on the model organism yeast rather than mammalian cells, as yeast vacuoles are similar to mammalian lysosomes but are very big (2-4 μm), making them easier to image. By tracking these yeast vacuoles they can be observed to fuse together or undergo fission into smaller pieces. These studies involve live cell imaging and long time-lapse imaging using spinning disk confocal microscopy.
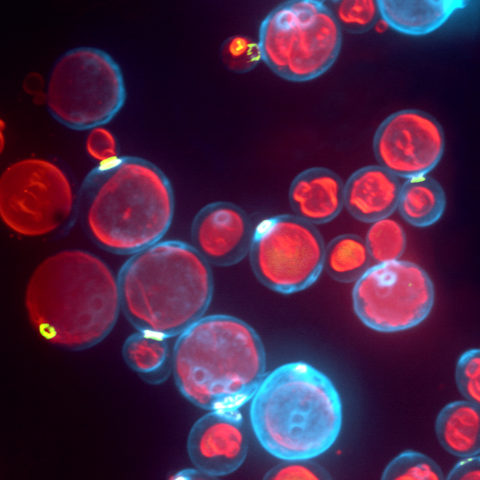
This represents a 3D reconstruction of a stack of confocal images taken on a spinning disk system.
Challenge
As Prof. Mayer states, “For us, a major challenge is photobleaching and phototoxicity because we want to look at the cells and organelles for a long time with repeated exposures… if we have low sensitivity we see many artifacts inside the cells, we get artificial fusion of organelles, purely light induced. These we can minimize if we have a microscope with maximum sensitivity.”
Most of these images are taken as stacks to build into 3D images over time. When imaging up to an hour, taking 10-20 slices per minute, there is an accumulation of exposure and phototoxicity, meaning that the exposure needs to be as short as possible, and the camera as sensitive as possible. A combination of speed and sensitivity would allow for minimal phototoxicity.
With the BSI we can definitely detect signals that we had a hard time detecting before, and do time courses now that were not doable before as the cells would be bleached or dying from phototoxicity by the end of the experiment.
Solution
Based on the need for sensitivity the Prime BSI is a clear choice, as outlined by Prof. Mayer, “We have mounted two Prime BSIs on our spinning disk system, and we appreciate the high sensitivity of the camera while it still gives a good framerate, which is more than sufficient for our purposes.”
The high sensitivity and speed of the BSI means minimal exposure times are an option, reducing the phototoxicity and allowing for long experiments. Prof. Mayer shared, “Now we can definitely detect signals that we had a hard time detecting before, signals now come out much clearer. We can do time courses now that were not doable before as the cells would be bleached or dying from phototoxicity by the end of the experiment.”