Prof. Ulrich Kubitscheck, Dr. Martin Schwarz, Mr Juan Eduardo Rodriguez-Gatica
Institute of Physical and Theoretical Chemistry, University of Bonn, Germany
Background
The lab of Prof. Ulrich Kubitscheck works to analyze living as well as cleared biological cell systems, developing novel quantitative light microscopy techniques. A combination of tissue clearing with light-sheet fluorescence microscopy (LSFM) is especially well suited to the fast analysis of complex arrangements of large cleared cell clusters and thus allows fast light microscopic access into the complex 3D architecture of neuronal tissue.
The limitations of LSFM and potential solutions to these were outlined by Prof. Kubitscheck: “LSFM cannot reveal the very fine details of neuronal networks since these structures are well below the optical diffraction limit and are of extreme complexity. A solution to this issue is realized by the combination of light-sheet fluorescence and expansion microscopy (LSFEM) allowing for the analysis of extended neural circuits in super-resolution… LSFEM is compatible with multicolor fluorescence imaging, thus enabling molecular contrast for diverse neuronal populations and nanoscale resolution within a single, large tissue preparation. LSFEM is optimally suited for the analysis of connectivity in large neuronal tissue regions.”
“Together with Dr. Martin Schwarz from the University of Bonn Medical School, we developed a novel tissue expansion pipeline and used LSFM and LSFEM to image very extended regions of mouse brain tissue in 3D. We can zoom in and out from meso- to nanoscale resolution, which means effective super-resolution, depicting the finest details of neuronal network parameters within a larger context.”
In this experiment, projections from the horizontal diagonal band of Broca (HDB) to the olfactory bulb (OB) in the mouse brain are visualized using LSFEM and a novel clearing protocol.
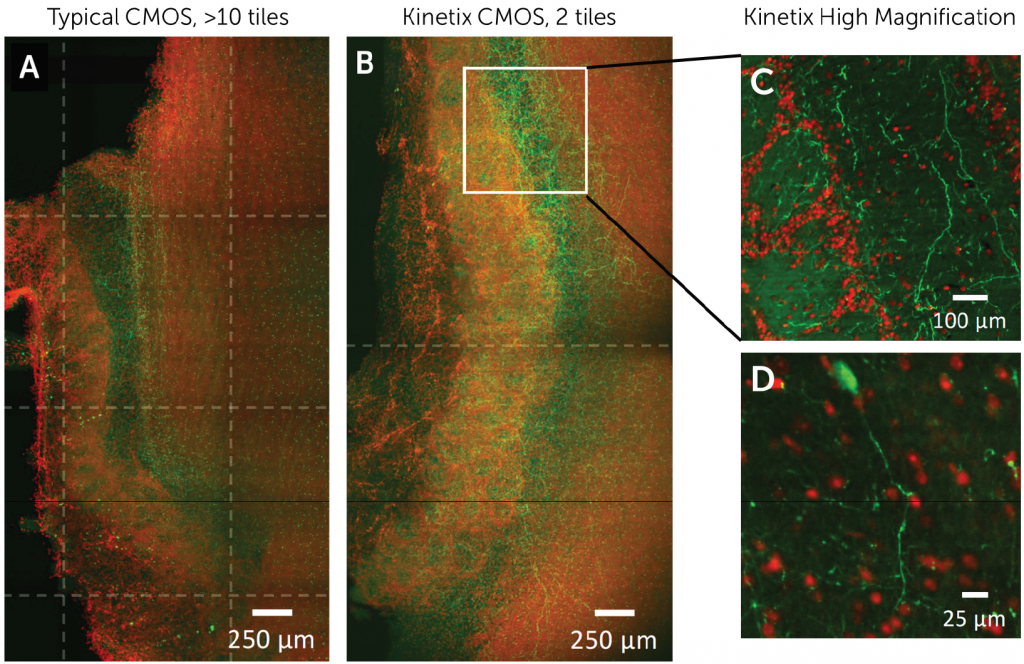
Challenge
LSFEM involves huge specimen regions and consequently, a large number of image tiles acquisitions are needed to cover the complete field. These images often need to be overlapping image fields to allow stitching, which adds to both the time taken for an image to be produced and the computational effort to perform stitching of a large number of image tiles. By using a camera with a very large field of view, a smaller number of acquisitions would be needed, reducing both the time for acquisition and processing.
The current imaging experiments can often have an exceedingly long duration, during which time the sample is exposed to light and risks photobleaching. A highly sensitive camera with a low noise level is needed in order to reduce the exposure required to image the sample and avoid photodamage.
Finally, a small pixel size is also required in order to achieve diffraction-limited resolution, this technique combines the needs of light sheet and super-resolution imaging, meaning the camera also needs to operate at a high resolution at certain magnifications.
The large sensor of the Kinetix greatly reduces the number of required image tiles to cover the field of view… the signal to noise ratio is excellent.
Solution
The groundbreaking Kinetix CMOS features a large field of view, a 10 megapixel sensor with a small 6.5 μm pixel size, and a high sensitivity thanks to a combination of back-illumination and low-noise imaging modes. This makes the Kinetix an ideal solution for this demanding imaging application.
Prof. Kubitscheck told us about his experience with the Kinetix CMOS, “As demonstrated, the large sensor of the Kinetix greatly reduces the number of required image tiles to cover the interesting field of view ranging from the HDB to the olfactory bulb area of a 1.5x expanded and cleared mouse brain section. The signal to noise ratio is excellent.”
“Using the new Kinetix camera in combination with novel tissue clearing protocols and LSFM we can show in great detail and overextended regions that HDB neurons innervate different cell layers of the OB. Most notably, we can, due to the large field of view, the exquisite resolution and the possibility to image ~2mm thick sections, follow projections from individual HDB neurons up to their target region within the OB.”
“The new setup comprising the Kinetix camera will significantly improve our imaging capabilities and will likely lead to novel insight into the HDB to OB synaptome.”
